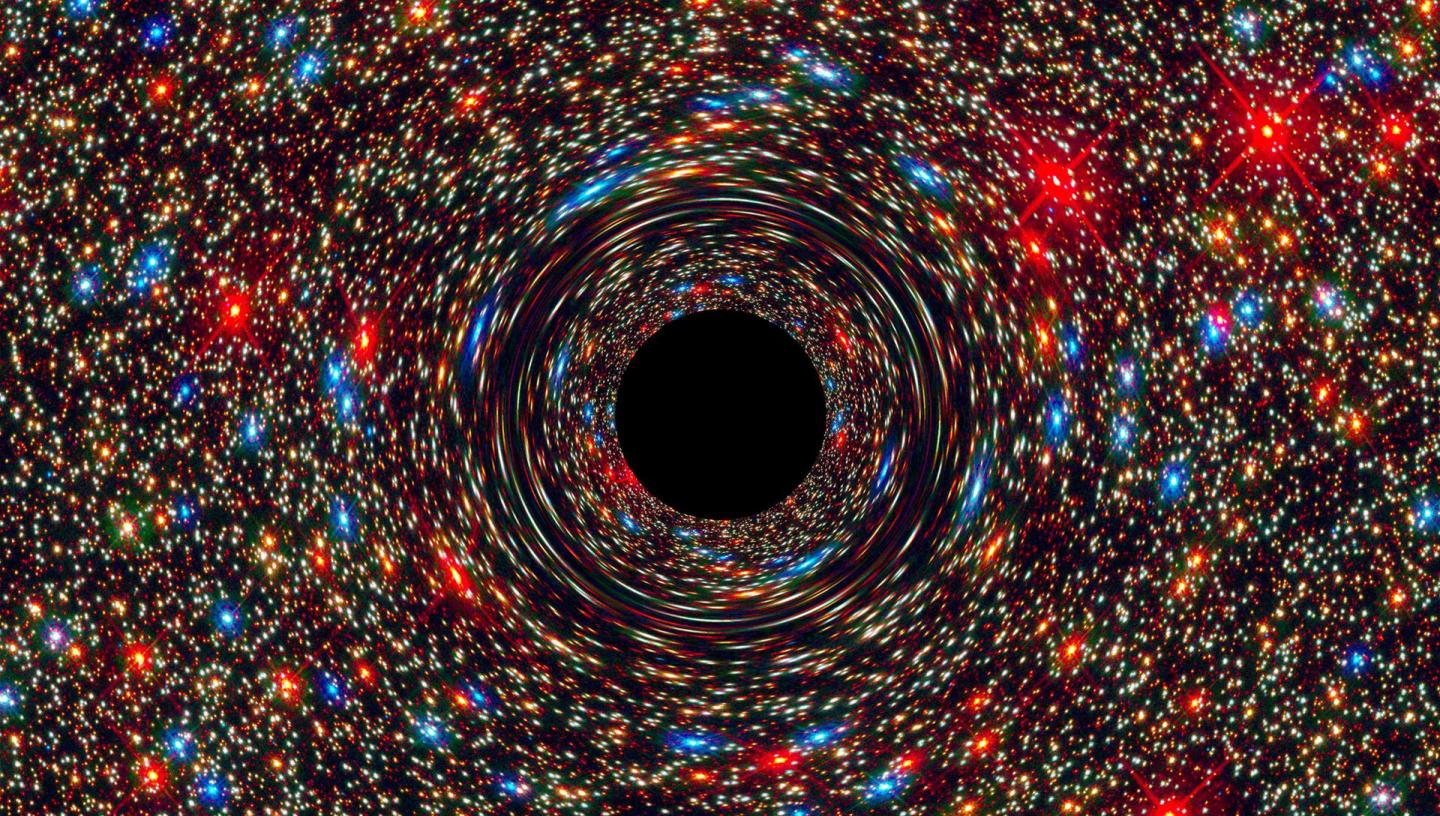
A black hole is a region of spacetime so curved by gravity that even light cannot escape it.
In his new book Black Holes, astronomer Dr Ed Bloomer explains in simple terms exactly how black holes are formed, what's inside them - and what would happen if you were ever unlucky enough to fall into one.
In this special extract, Ed describes the curious process of 'spaghettification'...
Falling into a black hole
One of the most common questions astronomers are asked is: what happens if I fall into a black hole?
Quite why it seems of genuine concern to so many people is beyond the scope of this article, but considering it seriously is a rather good way of making sense of exactly how black holes work.
Let’s say you’ve been exploring the galaxy and got a bit too close to a black hole without realising.
I, eminently more sensible, have stayed pretty far away on a distant space station, but I’m willing to help if I can! And, being generous as well as sensible, I gave you an amazing spaceship that can do almost anything. The question is, will almost be enough?
In our situation ‘too close’ means you can’t put yourself into a stable orbit, so you’re going to have to expend energy to maintain an orbit. You’ve crossed the innermost stable circular orbit, or ISCO. No problem, your spaceship has powerful and efficient engines.
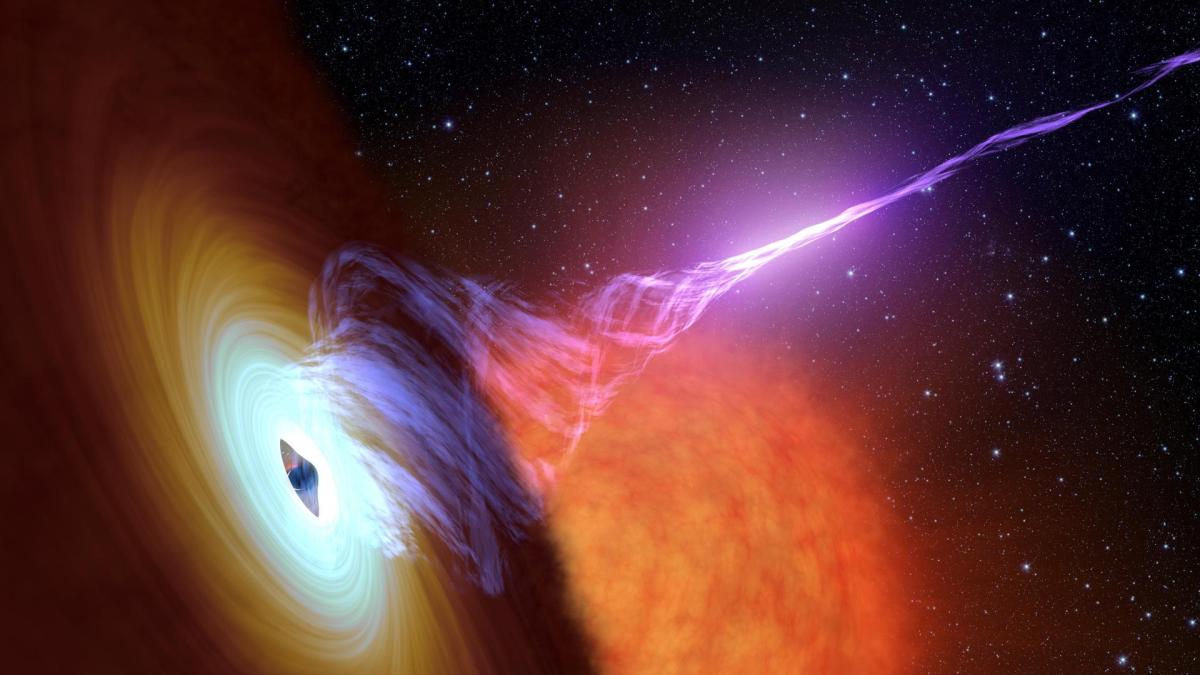
Alas, other stuff is drifting towards the black hole too. Molecules of gas, a bit of dust, perhaps some other unfortunates without a spaceship as good as yours. You’ve found yourself making up part of the accretion disc of infalling material, and that isn’t quite as collegiate as it sounds.
Though not part of the black hole itself, this material heats up due to friction and produces electromagnetic radiation and it falls further inwards.
The wavelength and intensity of the radiation depends on the mass of what you’re spiralling into – the accretion discs of protostars, for example, radiate in the infrared, which might not be too bad because that emission is quite low-energy. But in the case of black holes, the gravity and friction are sufficient to blast you with high-energy x-rays or gamma rays. Sorry!
Because the disc is flat, moving around what you’re going to think of as the equator of the black hole, you might try to get yourself ‘above’ or ‘below’ the disc, but you’ll be pulled back into alignment. You haven’t even reached the weird stuff – this is fairly conventional mechanics – though already you might realise you’re in trouble.
It’s probably time to start signalling for help, so you send a radio signal to the distant space station from where I’m observing things as best I can. Realistically, what can I do?
As you get closer to the black hole, the signals you’re sending are undergoing increased gravitational redshift as the photons climb out of the gravitational well. The space station is literally receiving different wavelengths than you’re transmitting, but while I’m busy retuning the receivers, you’re becoming ever more worried about the tidal forces acting on the spaceship.
The gravitational gradient as you get closer to the black hole is increasingly steep, so the difference between the gravitational pull on the parts of the spacecraft nearer the black hole and those further away grows.
You’re beginning to stretch, resisting that stretch by the strength of the material making up your spacecraft. But in extreme situations the tidal forces will pull you apart, a process known as spaghettification.
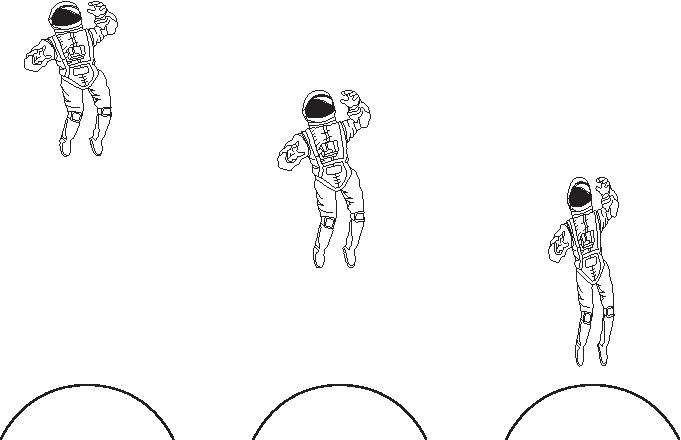
What is spaghettification?
In astrophysics, spaghettification is the tidal effect caused by strong gravitational fields. When falling towards a black hole, for example, an object is stretched in the direction of the black hole (and compressed perpendicular to it as it falls). In effect, the object can be distorted into a long, thin version of its undistorted shape, as though being stretched like spaghetti.
The curved line in the diagram represents a section of the surface of the black hole. In the left hand drawing, the astronaut’s height and width correspond as expected. As they move closer to the centre of the black hole, they experience slight compression horizontally and elongation vertically. In the right hand image, they are closer still and the compression and elongation of their form are even more dramatic.
Spaghettification is not inevitable. Black holes of different masses will have different gradients, so with supermassive black holes it is perfectly possible to pass the event horizon with no ill-effect. Again, this is not to say that the gravitational pull isn’t strong, just that the gradient isn’t too extreme. Let’s assume this is the case.
Unfortunately, other stuff seems to be falling into our black hole too.
Although a bit of company might seem welcome, infalling particles spiral into the black hole in a turbulent flow, rubbing up against each other. As we’ve seen, the accretion disc formed emits radiation due to this friction and, because of the immensity of the gravitational pull, particles are accelerated up to significant fractions of the speed of light.
The result is highly energetic radiation, including things like powerful x-rays. The black hole may even produce tightly focused astrophysical jets of ionised matter (sufficiently powerful and with velocities high enough to be referred to as relativistic jets).
Jet beams may extend millions of light years, so hopefully we would have noticed them before now. They’re also complicated things with unanswered questions surrounding them, so we won’t dwell on them too much. Significantly, they align with the axis of rotation, whereas we are approaching almost perpendicular to it in the accretion disc. You have enough to worry about, so let’s assume the jets aren’t a problem.
In fact, let’s assume you’re falling into an otherwise entirely quiet black hole and there are no jets or an accretion disc. Now the real fun begins.
(By the way, I’m happy enough to make all of these assumptions, but it is worth noting how many we’re making just so the ‘ordinary’ mechanics of a black hole don’t destroy us!)
The gravity of the black hole warps spacetime itself. In our case we’re interested in a process known as time dilation, another relativistic effect. It is a particularly complicated issue, so we’ll treat it with a light touch here. But even so, if we want to describe its effects, we have to be careful about our point of view. Things will look quite different to observers in different positions.
Let’s assume I am still in that distant space station you signalled for help. Time dilation means that from my perspective you actually start to slow down as you fall into the black hole. As far as I’m concerned, time is literally passing more slowly for you than for me.
By my calculations, your passage through time is going to crawl to a halt as you approach the event horizon.
Remember, I’m finding it increasingly hard to even detect you, since your radio signals for help and any other photons you’re emitting are being stretched to longer and longer wavelengths. You, meanwhile, are experiencing time pass normally.
Hold on, isn’t time… time? How can we be experiencing it so differently?
Well, time itself flows at different rates depending on what is happening. These aren’t figures of speech: objects move in spacetime at different rates (not just in the normal three dimensions associated with their velocity as we normally understand it, but in time too). We’re really noticing the effects because of the extreme situation we’ve engineered, but to a greater or lesser extent these distortions are happening wherever you are.
If everything else was fine, you’d simply pass the event horizon and keep going towards the singularity. Of course, the words ‘fine’ and ‘simply’ are doing a lot of work here!
What’s happening ‘outside’ the black hole while that’s going on? Well, sadly I’ve had to give up on being able to reach you or even get a signal from you. You’ve passed the point of no return.
From your point of view, there is no 'outside' to reach anymore, since every direction leads you towards the centre of the black hole. From my point of view and to all intents and purposes, you’ve ceased to exist.
This is why black holes are just... the end, both in space and time.
Effectively, things crossing the event horizon play no further part in the Universe. They cannot signal anyone and vice versa. And when we say signal, we don’t mean sending a coherent message. We simply mean transmitting any information at all.
Imagine you are an astronomer sitting in a laboratory beside an astonishingly sensitive detector that somehow reacts to a single, solitary photon of any wavelength emitted from beyond the event horizon of a black hole. When it does, it will set off an ear-splitting siren, so you definitely won’t fail to notice.
But day after day, year after year, there will be nothing but silence. As far as that detector is concerned, there is simply nothing there. A complete information void.
In summary: don’t go near black holes!
Black hole glossary – what do these terms mean?
Accretion disc A dynamic disc-like structure of material spiralling towards a massive object such as a black hole. Things like dust and gas molecules colliding or rubbing against each other as they spiral inwards might emit radiation, which can then be used to map out the spacetime near a black hole’s event horizon, though not inside it.
Gravitational redshift describes the process by which photons have to ‘climb out’ of a massive object’s gravitational well, losing energy and shifting to longer wavelengths. Thus, light emitted from an object will be received at different wavelengths (or frequencies) depending on the receiver’s position relative to the emitter.
Innermost Stable Circular Orbit (ISCO) Objects cannot maintain stable orbits at every distance from a black hole. The ISCO boundary marks the threshold at which orbits become unstable and objects move on a trajectory further towards the black hole. The position of the boundary depends on the black hole.
Main image courtesy of NASA Goddard