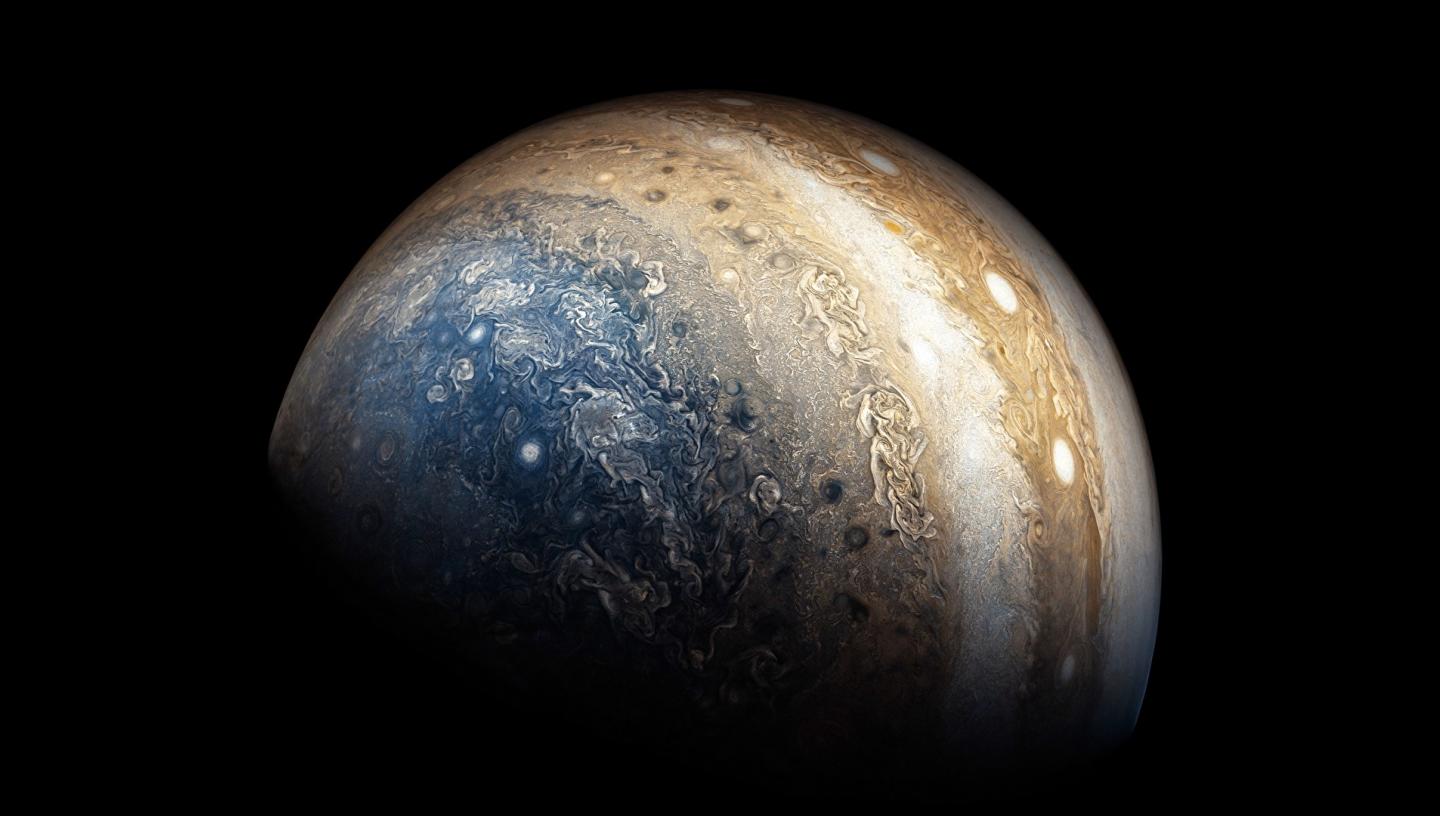
Juno is a space probe developed by NASA which is currently orbiting around Jupiter. Now 4 years into its mission, what has the Juno probe revealed about the largest planet in our solar system?
Why is it called Juno?
In roman mythology Jupiter was the king of the gods and the Juno probe is named after the Roman goddess Juno - the wife of Jupiter and the queen of the gods. Jupiter was a bit of a flirtatious character and had many lady friends that Juno was not pleased by. To hide his mischief, Jupiter drew a veil of clouds around himself, but Juno was able to lift the clouds and reveal his true nature.
Aptly named, the Juno probe’s mission is to reveal some of the secrets of Jupiter - notably how it originated and evolved which in turn may give us a better understanding of the solar system’s beginnings.
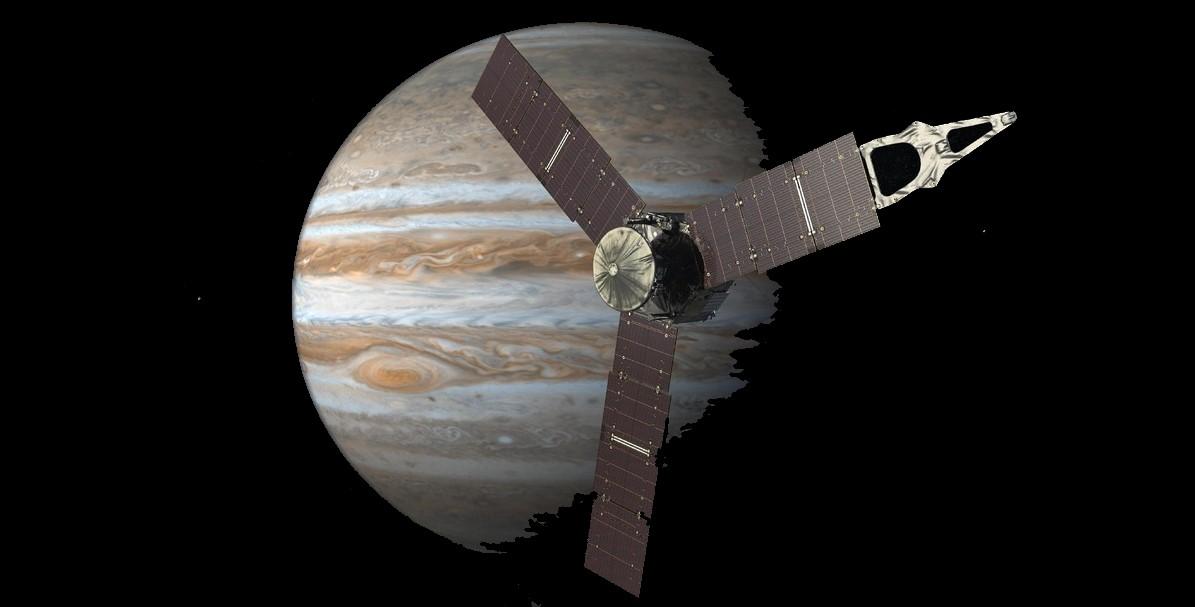
What are the main aims of the Juno mission?
- To work out how much water is in Jupiter’s atmosphere (something that will tell us which of the current planet formation theories is likely to be correct or highlight that we need new ones).
- To peer deep into Jupiter’s atmosphere to give us a better understanding of what it’s made of (composition), its temperature and its cloud motions amongst other things.
- To map the gravity field and magnetic field around this monster planet which will hint at what Jupiter’s deep structure is like.
- To explore Jupiter’s magnetosphere (the region surrounding the planet in which charged particles are affected by its magnetic field) especially near its poles. This will provide new insights into how Jupiter’s gigantic magnetic field affects its atmosphere and the creation of its aurorae.
Alternative mission objectives
Although this was a robotic mission, the Juno spacecraft did have some passengers. Onboard were three miniature Lego figures of Jupiter, Juno and the Italian astronomer Galileo Galilei - the discoverer of the four largest moons around Jupiter. Made of aluminium (a non-magnetic metal which wouldn’t interfere with the onboard equipment), these 4 cm figures were sent with an alternative mission in mind – to inspire young people to explore and develop an interest in STEM (Science, Technology, Engineering and Mathematics) and to encourage them to imagine and dream about journeying to the king of the planets ourselves, a feat that may seem impossible now but may one day become a reality.
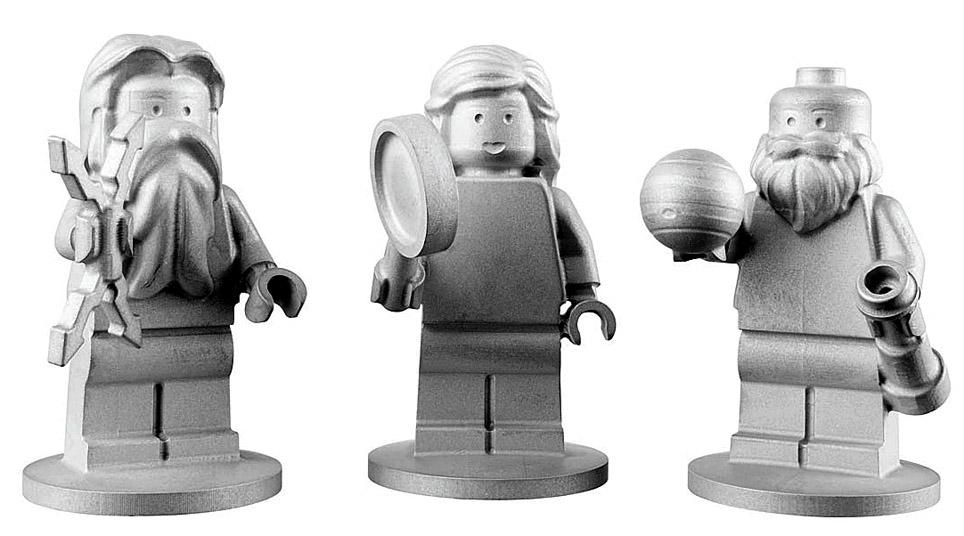
Launch, journey and arriving at Jupiter
The Juno probe was launched from Cape Canaveral Air Force Station on 5th August 2011 but the mission itself began back in 2005 when it was approved by NASA after several years of a strong desire for a Jupiter probe. The average distance to Jupiter is around 800 million km but the Juno probe travelled roughly 2.8 billion km to get there taking just under 5 years, due to a trajectory that used a gravity assist (speed boost) from the Earth. After the gravity assist in October 2013 which gave it a speed boost of more than 14,000 km/h, the probe headed for Jupiter. Accelerated by Jupiter’s gravity on approach, it arrived with a speed of around 210,000 km/h and so the spacecraft underwent insertion burns (firing of its engines) to decelerate it and finally entered Jupiter orbit on 5th July 2016.
The Juno probe has a highly elliptical polar orbit which means that its orbit is not perfectly circular around Jupiter and it is able to view Jupiter’s poles clearly (something that hasn’t been done before). The 53-day orbit brings it up close before taking it back far out from Jupiter and although it was intended for the probe to drop into a shorter 14-day orbit, instrumental problems that could jeopardise the engine burns required to achieve this meant that the scientists opted to keep Juno in its original 53-day orbit which is slowly moving northward, as intended. So with each orbital flyby, we’ll be able to see more and more of Jupiter’s northern hemisphere with better clarity.
Powering the Juno spacecraft
There has only been one other spacecraft that has orbited around Jupiter – the Galileo spacecraft, which operated between 1995 and 2003 (and included the Galileo entry probe that dived down into Jupiter’s atmosphere in 1995). The Galileo spacecraft was nuclear-powered alike to all spacecraft sent into the outer solar system at that time. So far from the Sun, it would be difficult to generate energy using solar power, but the limited nuclear fuel source put a restriction on its lifetime.
Juno is different – it’s the first spacecraft to be sent to the outer solar system that generates its power using solar arrays. Juno boasts the 3 largest solar array wings ever used on a planetary probe and not only are they necessary to generate the power needed to operate the spacecraft, they also help to stabilise it. Each of the solar arrays is 9m long (about the length of a bus) and together hold nearly 20,000 solar cells which could generate 14 kW of power when placed at the Earth’s distance from the Sun – that’s enough to power around 100 desktop computers! But Jupiter is five times further from the Sun compared to the Earth and the light that solar arrays would receive when that far out in the solar system would be 25 times less. But the probe is very efficiently designed and with only 500 W of power it’s still able to crack on with its work.
So what has Juno discovered in the time it’s been investigating Jupiter?
Mission aim 1: To work out how much water is in Jupiter’s atmosphere (something that will tell us which of the current planet formation theories is likely to be correct or highlight that we need new ones).
In February 2020, the first results (using data from the Juno mission) of the amount of water in Jupiter’s atmosphere was announced in a scientific paper published in the Nature Astronomy journal. It suggests that water makes up about 0.25% of the molecules in the atmosphere at Jupiter’s equator. Data from the Galileo probe from 1995 had suggested that Jupiter was much drier than the Sun (it was less abundant in oxygen and hydrogen – the elements that make up water) but these results from Juno show that Jupiter has almost three times as much water as the Sun does.
This is important for working out how Jupiter may have formed. The Sun was the first thing to be created in our solar system and from the disc of gas and dust surrounding it, planets began to form. Many scientists suggest Jupiter was the first planet to form accreting and pulling together material from the disc - it contains most of the gas and dust that didn’t go into forming the Sun. There are two leading theories as to how Jupiter came to be: one is that Jupiter formed pretty much where it is now and the other theory suggests it formed further out in the solar system and has since migrated in to its current location.
If Jupiter formed at its current location from the accretion of material in the solar nebula, then only some elements would have been in a solid phase but other heavier elements like oxygen and nitrogen would have still been volatile – they would have easily evaporated and so dispersed. But if Jupiter formed further out in the colder depths of the solar system, these heavier volatile elements could freeze and therefore accrete and join together.
Because water contains oxygen and the abundance of oxygen is linked to where the planet may have formed, these results could help sort this dispute.
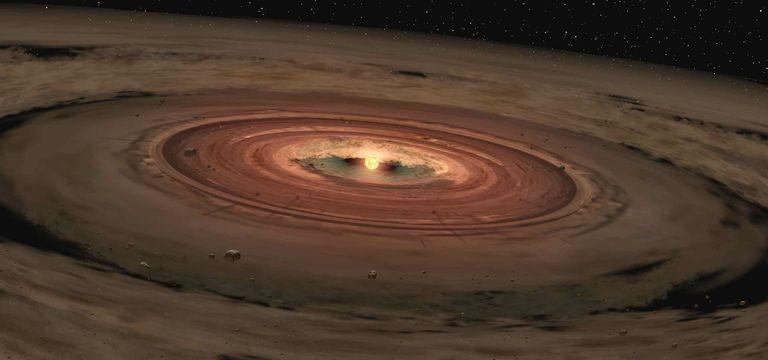
But scientists have noted that Jupiter’s equatorial regions are quite unique - it’s the only areas where everything seems to be well mixed. When you move north or south from there it’s not the same case. So these results need to be compared with how much water is in other regions. The atmosphere doesn’t appear to be well mixed and the amount of water might vary across the planet. This is perhaps why data from the Galileo probe hadn’t suggested the same thing – the probe just so happened to fall into a particularly dry spot on Jupiter.
Mission aim 2: To peer deep into Jupiter’s atmosphere to give us a better understanding of what it’s made of (composition), its temperature and its cloud motions amongst other things.
Jupiter is a massive planet – it contains a lot of material and the more mass there is, the stronger the gravity. Juno has an instrument that can measure the gravity field around Jupiter which gives an indication of its mass and when combined with measurements of its size this can give scientists an estimate of the density of Jupiter (how tightly packed the material in this planet is). Knowing its density can give us an idea of its composition – what it must be made of (mostly lighter elements or heavy elements).
By mass, Jupiter’s atmosphere is composed of approximately 75% hydrogen and 24% helium with the remaining percent consisting of other elements. There are trace amounts of methane, water vapour, ammonia, and silicon-based compounds as well as carbon, ethane, hydrogen sulphide, neon, oxygen, phosphine, and sulphur. Deeper in its interior, it contains denser materials so its composition changes slightly.
Jupiter is marked with alternating bands of gas which are known as belts and zones – something that the astronomer Galileo observed himself over 400 years ago. The bands are cloud features along Jupiter’s jets - incredibly strong winds blowing around the entire planet at around 360 km/h. The winds in the dark coloured belts flow in one direction and the winds flow in the opposite direction in the brighter zones. Just like on Earth, clouds vary in altitude / height. On Jupiter, the cloud tops are higher in a belt than in a zone. How deep these colourful bands and other features penetrate down into the atmosphere of Jupiter is one of the key questions that scientists are looking to find the answer to.
For a solid body the gravity field around it is symmetrical but in a body that has internal dynamics i.e. is made of liquid or gas so changes and isn’t fixed, the gravity field can have asymmetries and it's attributed to differential rotation (that some parts of it spin / rotate quicker than other parts of it do) and is also the result of deep atmospheric flows. Juno has found that the gravity field around Jupiter varies from pole to pole. The deeper the jets, the more mass they must contain which would lead to a stronger signal in the gravity field. So by studying the gravity field, scientist were able to determine just how deep these jet streams penetrate beneath the visible clouds. Juno data indicated that this weather layer of Jupiter runs much deeper than expected and is more massive than predicted - reaching depths of 3000 km. The atmospheric winds on Jupiter extend deep into its atmosphere and they last a lot longer than similar atmospheric processes that we observe on Earth.
Juno’s Microwave Radiometer (MWR) is able to measure the atmospheric temperature at different depths by virtue of water and ammonia being good absorbers of certain wavelengths of microwave radiation. It’s the process by which our microwave ovens work – water molecules in our food absorb the energy from the microwaves, which heats the food quickly. Temperature measurements therefore give an indication of the amount of water and ammonia deep in Jupiter’s atmosphere. The MWR data has indicated that the belt near the equator penetrates all the way down (from the top of the ammonia clouds to deep within its atmosphere), while the belts and zones at other latitudes seem to evolve into other structures. The Galileo probe sent back data from depths of 120km before it stopped transmitting – at those depths, it experienced pressures of roughly 22 times the atmospheric pressure on Earth. But the sensors on Juno can measure the temperature and therefore the water content at depths where the pressure is 50 times greater than the Galileo probe experienced.
Juno’s polar orbit provides spectacular views of its poles and JunoCam (the camera instrument originally intended as an outreach tool) has taken some truly stunning images of Earth-sized swirling storms, densely clustered together at the north and south poles of Jupiter. At the north pole, there are eight storms encircling a single polar cyclone and in the south, five others arranged around a central storm were detected early on. But on a more recent flyby, Juno detected another which sprouted up in the south. These storms remain a mystery to scientists in terms of how they formed, why the storms remain in a stable configuration and don’t seem to interrupt each other as they rub side by side and why they don’t look the same at both poles. Over the remainder of the mission, Juno will continue to watch these storms to see if they persist or whether they disappear.
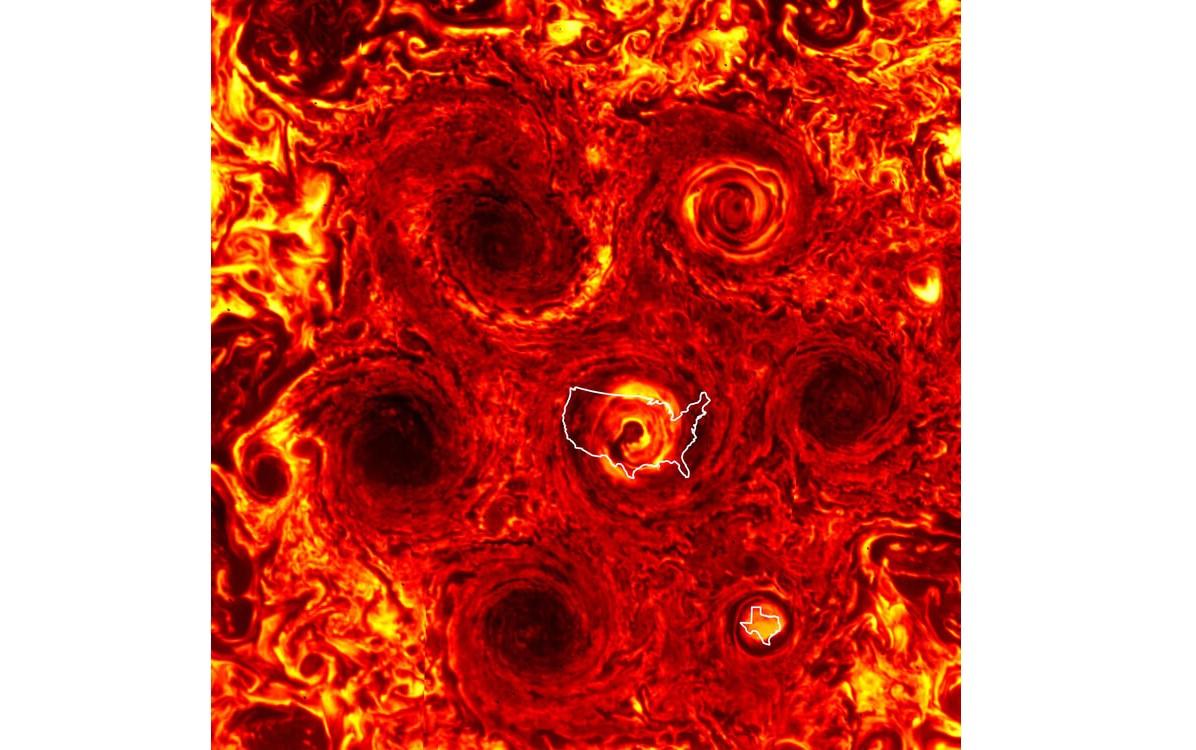
The Great Red Spot is perhaps Jupiter’s greatest feature – it’s a giant raging storm on its surface. Although scientists refer to it as a storm, technically it is an anticyclone. Alike to cyclones and hurricanes on Earth, the centre is relatively calm but at the periphery, winds soar between 430 to 680 km/h.
Whilst it has swirled across Jupiter for at least 200 years (that’s how long written records of its size have been collected), it may actually be more like 350 years old if early observations of a similar storm are indeed of the Great Red Spot itself. But this enormous feature has been slowing shrinking – records of its size showed it was getting smaller in the 19th century and again when NASA’s voyager probes flew by in 1979 (at that time it was over twice the width of the Earth). But Juno has revealed its closer to one and a half times the width of the Earth now. This shrinking sped up noticeably in May 2019 and blades or flakes (fragments of the storm) have been seen breaking off the main storm fizzling into the surrounding regions, and streaks of dark material have also been observed flowing from the Spot – making it appear as though the storm is unravelling. So astronomers, both professional and amateur, are watching avidly.
The storms on Jupiter are more persistent than similar phenomena on Earth. Hurricanes grow over oceans and when they reach land, they dissipate because the land acts to slow the storms. But since Jupiter has no surface as such, the winds can last for centuries – sometimes they even merge to form larger features or just gobble up smaller neighbouring storms that get too close.
It’s thought that the Great Red Spot is an area of upwelling, where clouds billow up from below. Some storms are white but many take on the brick-red colour like this storm. The exact chemistry and composition responsible for the colour remains to be determined but the main cloud layer of the Great Red Spot is likely ammonia, so that might be a factor.
Although we can see its width and measure its size, scientists have been eager to find out how deep this storm penetrates. Juno has been able to reveal that that Great Spot has roots that go about 300km down into its atmosphere – that’s 50 to 100 times deeper than Earth’s oceans. What’s more is the base of the storm is warmer than at the top and since winds are associated with differences in temperature (warm air rises and cold air sinks), the warmth at the base of the Great Red Spot goes some way to explaining the wild winds that are seen at the top of the atmosphere.
Mission aim 3: To map the gravity field and magnetic field around this monster planet which will hint at what Jupiter’s deep structure is like.
One of the instruments on board Juno is the gravity instrument which measures the gravitational pull from Jupiter on the spacecraft as it orbits around. So if Juno feels a stronger tug while flying over one region, it can infer that there’s something more massive or a dense region below the visible clouds that it’s flying over. Now that Juno has made enough orbits around Jupiter to build a global image of it, the gravity data can be used to build up a density map of this gas giant and begin to reveal what’s hidden beneath.
There are the two leading theories for Jupiter’s formation – that rocky debris slowly coalesced to form a solid core which then grew large enough that its gravitational pull swept up the lighter hydrogen and helium gas into a huge envelope around it and the other idea is that Jupiter was born from a dense pocket of gas that was left swirling around the Sun and it collapsed in on itself without a rocky core. Because we can’t peer right into the centre of Jupiter, its internal structure is something that has eluded scientist for decades.
But once again Juno has made headway with this. From being sped up or slowed down by Jupiter’s gravity over different regions, scientists have been able to track the mass distribution around the planet using the gravity instrument. The data suggests that Jupiter has a core but not as expected. It’s not a compact ball at the centre with a clearly defined edge, instead it is a fuzzy diluted core which spreads across almost half of Jupiter’s 143,000 km diameter. The reason for this is still a mystery though some have proposed that it may have been struck in its early life which ended up mixing material from the core with the hydrogen-helium envelope that fills much of the rest of the planet. So Jupiter is unlikely to have a solid core but as the gas from the upper layers gets squashed and contracted in the layers beneath, the soaring temperatures and crushing pressures are likely to transform the gas into something a bit more exotic – liquid metallic hydrogen (alike to the mercury found in old thermometers). The temperature in the clouds of Jupiter is about minus 145 degrees Celsius but near the planet's centre, it’s much hotter - it could be around 24,000 degrees Celsius, which would make it hotter than the surface of the Sun.
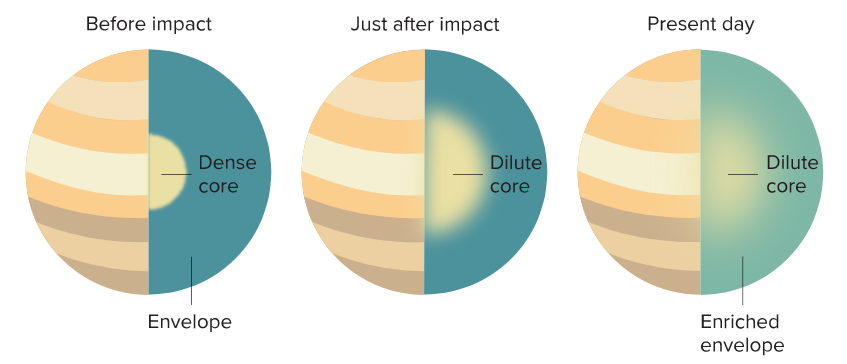
The internal structure of Jupiter has impacts on other aspects of its behaviours. Prior to the Juno mission, scientists were well aware that Jupiter had an incredibly intense magnetic field, but the results from Juno’s magnetometer instrument has shown it’s more irregular and stronger than anyone had expected – about 10 times stronger than the strongest magnetic field found on Earth.
The spacecraft magnetometer, which is mapping the gas giant’s great magnetic field found this striking result on its first orbit around Jupiter and now that enough data has been gathered to provide global coverage and map the planet’s magnetic field – something very strange has emerged. The magnetic field is lumpy and uneven – stronger in some place than others. The Earth’s magnetic field is produced by a dynamo. Surrounding the Earth’s solid core is an electrically conducting fluid – liquid iron. The rotation of this fluid produces our planet’s magnetic field. Our planet has two poles – the north and south magnetic poles with field lines that resemble that around a bar magnet - so it's like there's a bar magnet within the Earth. But Jupiter’s magnetic field is a bit of a mess and that’s an understatement. Imagine taking a bar magnet, bending it so it’s not completely straight, then fraying one end of it and spliting the other end in two before placing it in the planet at an obscure angle. In the north, the magnetic field lines sprout like weeds rather than from around a central point (that’s the frayed end) and then in the south - some field lines converge and enter the planet around its south pole but some gather back into the planet in a region just south of the equator – which has now been named the Great Blue Spot (slightly confusing because it’s not a storm). The unevenness of Jupiter’s magnetic field suggests that the magnetic field may be generated closer to the surface by a dynamo action rather than from deep within, like the Earth.
Mission aim 4: To explore Jupiter’s magnetosphere (the region surrounding the planet in which charged particles are affected by its magnetic field) especially near its poles. This will provide new insights into how Jupiter’s gigantic magnetic field affects its atmosphere and the creation of its aurorae.
Although first spotted by the Voyager 1 spacecraft, Juno’s polar orbits makes it perfect for studying the most powerful northern lights in the solar system. They cover huge regions of the planet's poles and are hundreds of times more energetic than aurorae on Earth. Juno’s polar orbit has also made it possible to capture views of Jupiter’s southern aurorae which are difficult to see from Earth due to the angle of Jupiter from our Earth bound view.
Aurorae are caused by accelerated charged particles colliding with atoms in the atmosphere of a planet which then release energy in the form of light. On Earth, it is charged particles from the Sun (the solar wind) that interact with the atoms in the Earth’s atmosphere and result in the spectacular light show that the aurorae are. The charged particles are driven to the poles of the planet by the magnetic field of the Earth and the resulting light show appears in visible light. Jupiter’s aurorae are the same fundamental phenomena however they are not only caused by charged particles from the Sun, but also from one of its moons – Io. But Jupiter’s aurorae shine brightly in ultraviolet and x-rays (and even other light) rather than visible light so we wouldn’t be able to see it with our eyes.
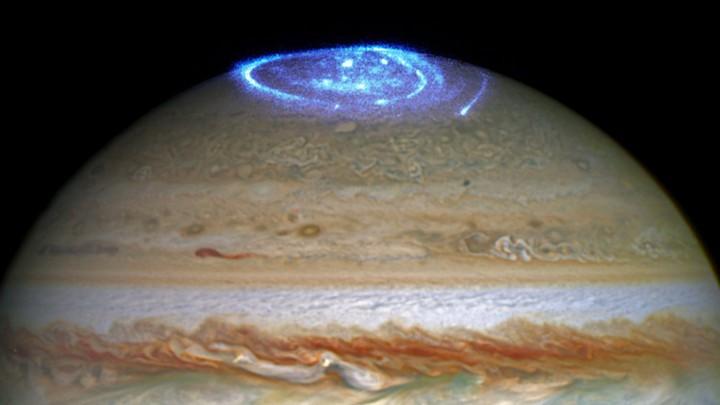
But once in a while, the aurorae grow to an incredible intensity and it’s not from a giant solar flare. Io is a volcanically active moon. The volcanism is the result of gravitational tugging on its surface and interior, not only from Jupiter but from the other large Galilean moons orbiting close by too. This tugging or tidal flexing heats up the interior of Io driving a series of volcanoes to erupt on its surface. Io ejects huge amount of sulphur dioxide and oxygen gas into space and this material becomes ionised or charged by Jupiter’s magnetic field and forms a doughnut-shaped track or torus around Io's orbit called the Io Plasma Torus. Over time the particles in the magnetosphere interact with the Jovian atmosphere to produce aurorae but on occasion there are also bright spots in the aurorae from charged particles flowing directly from Io towards Jupiter’s atmosphere.
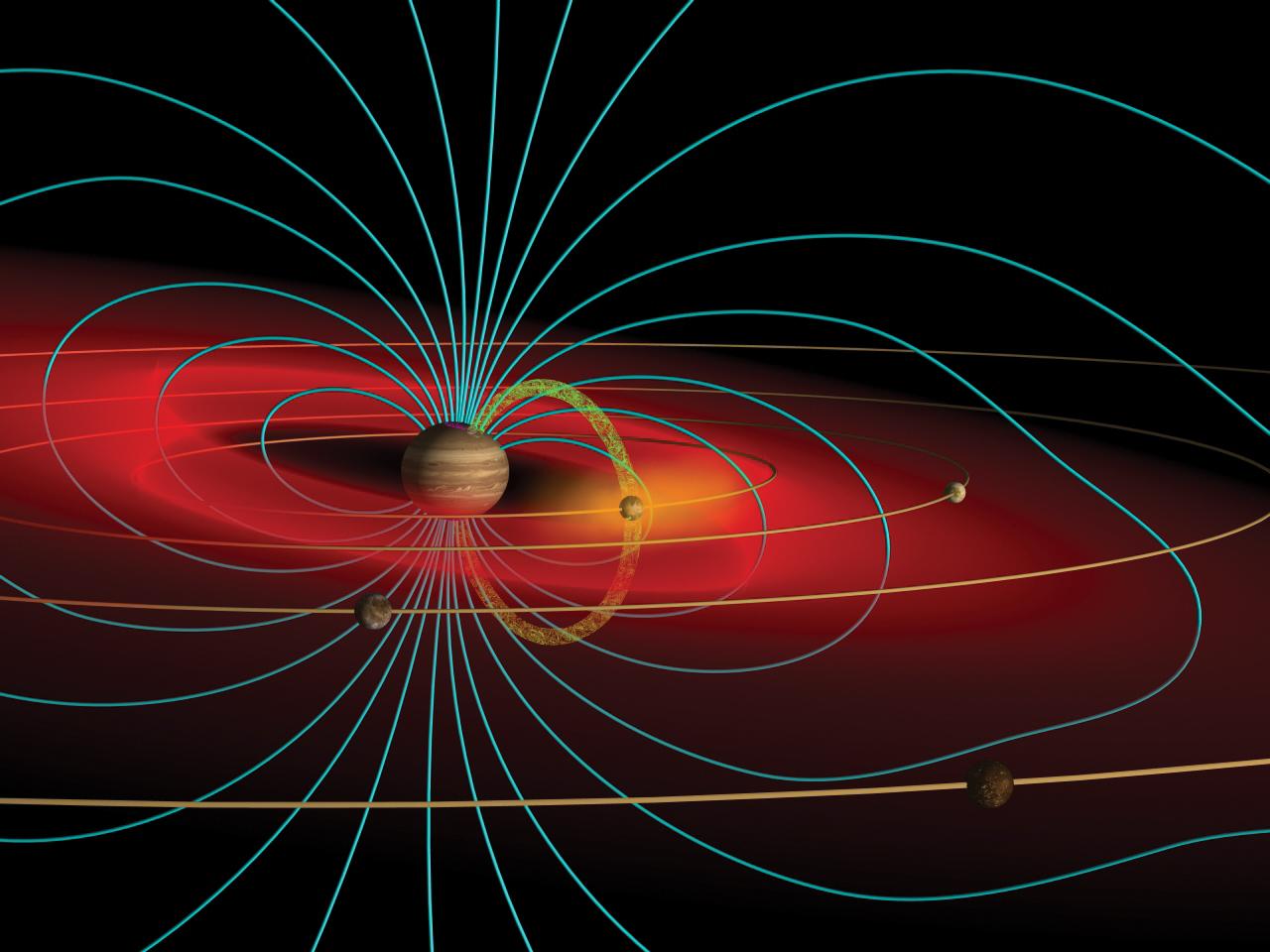
Jupiter’s large magnetic field is a result of its speedy rotation. Even though Jupiter is ten times wider than the Earth, it manages to spin on its axis two and a half times faster, making a full revolution in less than 10 hours. As Juno has started to uncover, the magnetic field is not generated by Jupiter’s core but rather its metallic hydrogen layer that’s closer to the surface. Jupiter’s magnetosphere (the region of space around it which affects charged particles) is bigger than the Sun! And due to its incredibly strong magnetic field, the aurorae on Jupiter never stop. The super quick rotation is what is thought to accelerate the charged particles into Jupiter’s atmosphere more strongly with a greater kick.
Because aurorae on Earth and Jupiter are the same phenomena, scientists expected that the charged particles that smash into Jupiter’s atmosphere would get their energy in the same way that the charged particles colliding with the Earth’s atmosphere do. That is, when charged particles spiral around the magnetic field lines of a planet, they create electric currents in the space above the atmosphere so when charged particles travel through that space they get an energy bump and are accelerated - it’s when these accelerated particles collide with an atom in the atmosphere, that the most energetic aurorae are produced. But Juno has deduced that this same mechanism is not responsible for producing ALL of the intense aurorae on Jupiter. On its first flyby over the aurorae it didn’t detect the charged particles being fired at Jupiter’s atmosphere with that greater kick as expected, but in subsequent flybys it did.
One possible explanation is that just above the atmosphere of Jupiter is a plasma region (a region of ionised or charged particles) and like surfers on ocean waves, particles can be accelerated by waves in the plasma. Slowly, by interacting with many waves, the particles gain energy and those that gain the most energy could be the other cause of Jupiter’s intense aurorae but it’s still a hypothesis as researchers haven’t worked out how those plasma waves could be generated.
The future of Juno
In June 2012, NASA extended the mission lifetime until July 2021. Juno’s highly elliptical orbit which would bring it close to the planet for only a few hours of its 53-day orbit was to limit constant radiation exposure to protect the sensitive components on the spacecraft from being destroyed. It was only expected to last for a few close passes but it's still flying strong today! The longer 53-day orbit means that it will take more time to collect the science data needed and although it has an unlimited fuel source as it’s solar powered, the mission’s overall duration will probably be limited by budget rather than the exposure to radiation from travelling through Jupiter’s magnetosphere (as its being doing well so far).
In its final planned year of orbiting around Jupiter, Juno will continue to delve deeper into the mysteries of Jupiter and help provide more in-depth answers to its mission aims. Halfway into its mission it completed global coverage of Jupiter albeit in coarse detail but in the remaining months it will complete orbits that have taken it halfway between those previous orbits which means it will be able provide an even more detailed picture of the entire planet. If the probe remains healthy there may be a chance of extending it past the end of its prime mission but the status of the spacecrafts instruments will need to be monitored to minimise the risk of an undesired collision with Jupiter’s moons should the spacecraft fail to operate as required and of course budgets will need to be reviewed.
At the end of the mission NASA plans to deorbit the Juno spacecraft sending it diving down into the atmosphere of Jupiter – alike to what the Galileo probe did back in 1995. When it made its dangerous descent, the Galileo probe was struck by winds of 640 km/h and was heated to temperatures double that experienced on the surface of the Sun – that’s what’s in store for Juno when it screams into Jupiter’s atmosphere. The deorbit is planned for 30th July 2021 and by controlling the spacecraft upon its demise, it will ensure the spacecraft is operated in accordance with NASA’s Planetary Protection Guidelines so not to remain as space debris or risk contamination by colliding into other celestial bodies since it will likely disintegrate as it submerges deeper into Jupiter’s atmosphere. But, during its final moments it will no doubt provide much anticipated and crucial data on the interior of Jupiter.
Written by Dhara Patel, Astronomy Education Officer (June 2020)